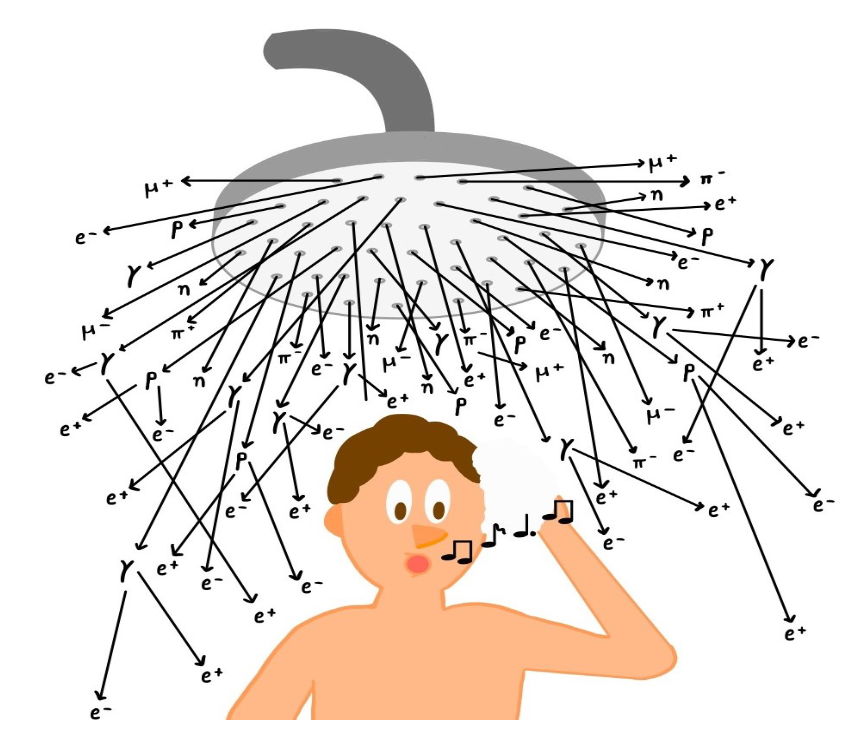
For most people, a shower is the feeling of warm water droplets falling on your skin, creating a relaxing start to the day. What most people don’t realise is that you are technically showering throughout the hours that you are awake, and even when you are asleep. Most of the time, however, it is not water that pours onto you, but particles. And these particles do not cleanse your skin – they do not even hit it. Crazy, right? It may sound far-fetched and almost made up. You have probably never noticed anything of the kind, but still, it is reality. So, you might be wondering, what are these particles and what does ‘showering’ in particles mean? Let me explain.
Particles from space
The above-mentioned particles are so small that our eyes cannot register them, which makes them invisible to us. They are even smaller than molecules. The cosmos is filled with these teeny tiny particles, where they fly around in the vacuum of outer space. Sometimes, a planet like Earth will cross their path and the particles collide with its atmosphere. This atmosphere, contrary to the cosmos, is filled with air and comprises the outermost layer surrounding the planet. It might not seem like it, but air consists of many molecules mixed together. At sea level, the air is of order 1026 times denser than the vacuum of the cosmos, which is an unfathomable difference.
The image above shows a fictional representation of the result of these particles colliding with the air in our atmosphere. Rather than droplets of particles, we are talking about cosmic rays and gamma rays. Cosmic rays are high-energy particles in, for example, the form of electrons, protons, or nuclei, that travel through space at nearly the speed of light. Gamma rays are similar but consist of photons hitting Earth’s atmosphere.
Two heads are better than one
The molecules in the atmosphere are exactly what cause the air showers. The particles can travel unhindered in space, but want to interact once they encounter all the matter in our atmosphere. This interaction can be seen as the particle hitting an air molecule. There is an important condition for a particle to interact: its energy must be high enough to do so. A high-energy particle is fast and carries a lot of momentum. When it collides with an air molecule, a more ‘intense’ reaction can take place than slower particles could cause. For cosmic rays, the reaction that ensues is complex and consists mostly of ionisation, electromagnetic, and hadronic interactions. How these reactions take place is complex, but the result is a cascade of many different particles and radiations, like for example muons, protons, antiprotons, alpha particles, pions, x-rays, electrons, positrons, and neutrons.
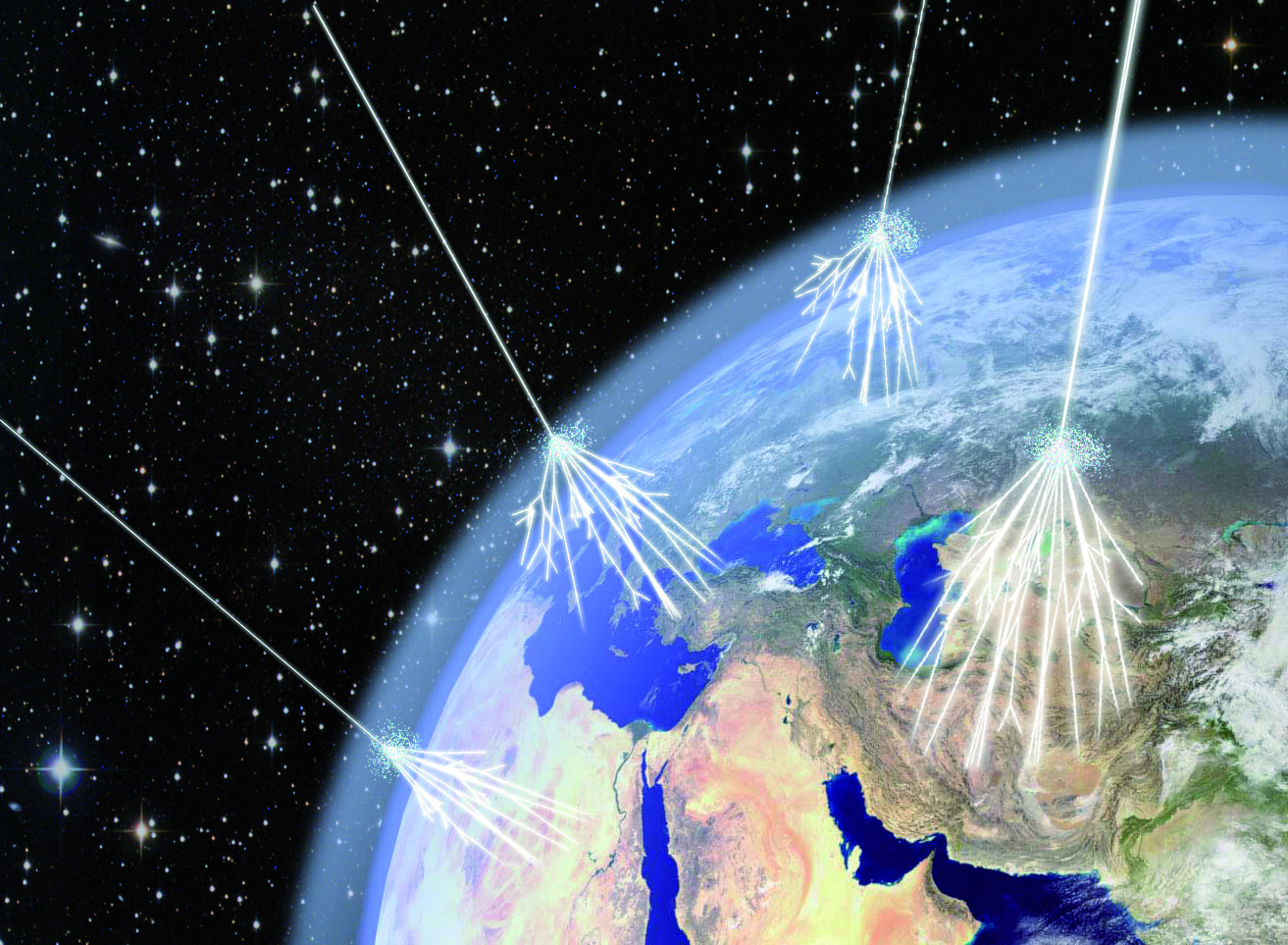
The interaction for photons – that is: gamma rays – is different and mostly results in pair production. This means in this case that one high-energy photon will convert into an electron-positron pair. One particle becomes two, which both carry a fraction of the energy of the original particle. No energy is lost in the reaction, which means that the energies of the two resulting particles, added together, give back the original energy. Both these resulting particles then also interact individually with matter via pair production, if they have a high enough energy. They convert into a pair themselves. This process repeats itself until the energy of the original particle is so ‘divided’ that it is too low to form a new pair. The result is seen in the image below.
Looking at the illustration, one can imagine why this process is called a shower. At the end of one shower, the original particle can have turned into millions or even billions of particles. Since high-energy photons, electrons, etc. are constantly entering our atmosphere, we are continuously standing underneath these millions of incoming particles. Showering throughout the day. Still, you might think: how do we know that these showers exist if we cannot see or feel them?
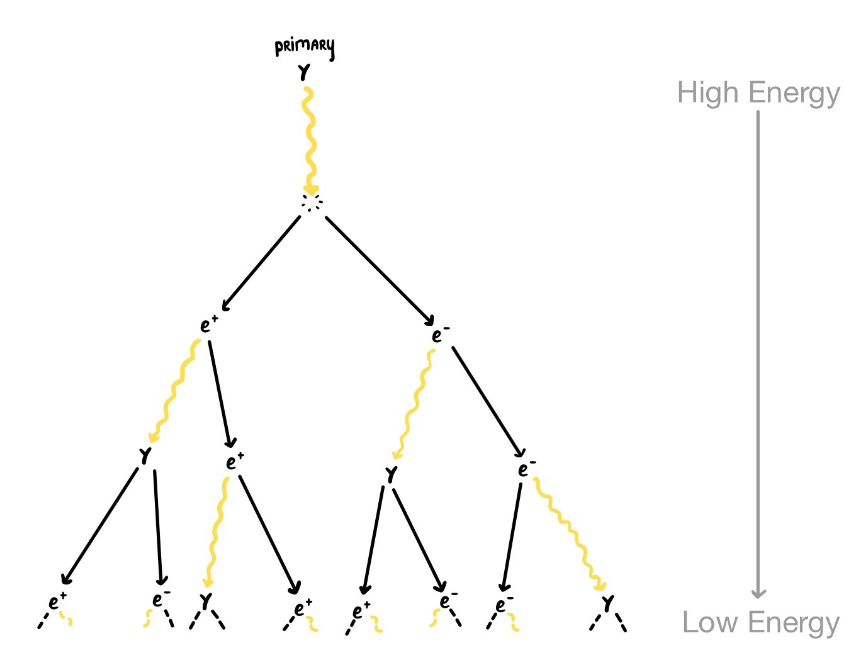
Some history
If you ask yourself the above question, you are right: for the longest time air showers were only a concept. In the first half of the nineteenth century, people were breaking their heads over results from ionisation measurements. At the time it was thought that only radiation from radioactive elements originating in the earth’s crust was able to ionise the air. However, the measurements showed that the amount of ionisation increased, rather than decreased, at higher altitudes. Soon, scientists started hypothesising the idea of a particle coming from space to ionise the air high up in the sky. It was Robert Millikan who came up with the term cosmic rays in the 1920s to explain this phenomenon.
It was not until 1938 that extensive air showers were first detected by Pierre Auger and his collaborators. Then, in 1954, the Rossi Cosmic Ray Group at MIT researched the properties of cosmic rays and elucidated these mysterious showers even further. Later, in 1967, the first gamma rays were tracked by a satellite of the same institute. It was only then that we were able to see them for the first time.
The detector
Researchers did not see the particles with their naked eyes, of course – they built a machine that could see them for us. Such a machine is called a detector, as it detects the particles from the shower. Over the years we have evolved our methods of detecting the air showers. One of the biggest detectors at present is the Pierre Auger Observatory in Argentina. However, even this machine cannot pick up on the invisible particles directly, so we have found smart ways to still know that they are there. These smart ways can be categorised into two complementary observation methods.
Fluorescence
The simpler-to-explain observation method is the detection of fluorescence. The particles in the air shower interact with nitrogen atoms in the atmosphere, emitting UV light via a process called fluorescence. We humans cannot see this UV light, because our eyes are not sensitive enough. However, the optical detectors in observatories are far more sensitive and can track the trail of light that these showers leave behind. Since at the bottom of the air shower or cascade, there are far more particles than at the top, the bottom of this shower is the brightest location. There, the detector can ‘see’ (in the above sense) the shower perfectly.
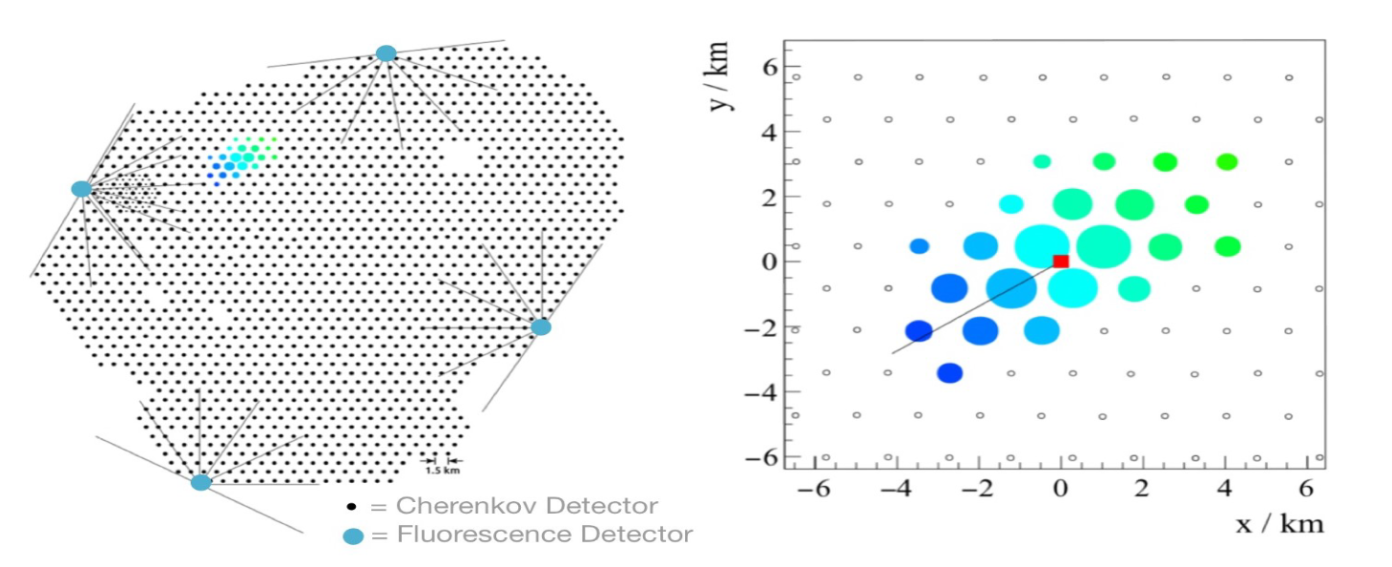
Cherenkov radiation
The second smart way can be summarised by the term ‘Cherenkov radiation’, which we can observe as a blue shining light. The high-energy particles of a cosmic shower travel incredibly fast – so fast that they approach the speed of light in a vacuum. The specification of ‘in a vacuum’ is needed, since the speed of light is not constant in all media or substances. In the vacuum, there are barely any molecules. Thus, light can travel unhindered and can reach its maximum velocity. In a denser substance like water, with a larger refractive index, the speed of light is lower. This can be visualised as the process that happens when crossing a street. If the street is empty, you can walk to the other side in one straight line, unhindered. If the street is buzzing with people, you need to zigzag your way through the masses, interacting with people, to reach the other side. The latter of the two examples will probably take longer. The same can be said for light in a denser medium. If there are more molecules, light has a harder time getting through and will slow down.
Light consists of photons, which have no charge. However, most of the high-energy particles in our air showers do have a charge, and this is where an important difference with our example arises. The charge can be seen as a circle of bodyguards around you when crossing the street. They take care of everyone in your way, so that regardless of how many people there are in the street, you can cross it at the nearly same pace. Thus, a charged high-energy particle will not slow down much in a medium denser than the vacuum. It will still travel at a speed very close to the vacuum speed of light.
This is where the story becomes interesting. Knowing this, we can imagine that in a dense medium, like for example water or air, our high-energy particles can travel faster than the local speed of light. This may sound crazy, particles travelling faster than light, but it is true. When this happens, the particles will emit a beautiful blue glow called ‘Cherenkov radiation’. The light will be emitted in a cone shape, due to the particle being faster than the light. Why a cone shape? Due to the particle’s velocity exceeding that of light in the medium, the emitted light cannot propagate ahead of the particle. Instead, it is emitted backward in an angle of about 45 degrees relative to the particle’s path. This emission angle is a consequence of the particle outrunning the light it emits.
Since the particles in cosmic showers are tiny and the air is not that dense, the light from Cherenkov radiation will be very faint, too faint for us to see if there are only a few particles. That is, once again, where the detectors come in. They have a much higher sensitivity than our eyes and can even register one singular light-particle or photon. In the image above you can see the results of one of these measurements. The bigger coloured dots are detectors that detect more Cherenkov light. The colour scale is representative of the timing of the different detections, the dark blue being triggered first and the green last. The image shows how the cosmic ray can then be ‘seen’. Instead of directly seeing the particles, the detector reconstructs where there are more particles by the amount of Cherenkov photons that are detected. That way, the paths can be reconstructed (the black line in the image), which can give rise to beautiful simulations of the actual event.
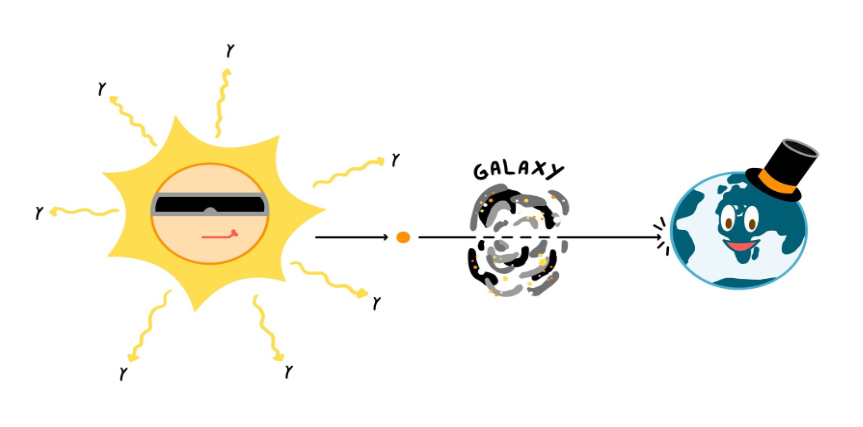
A final message
In conclusion, particles raining down on you is a fun and cool idea to think about. But why would we be interested in any of this? These high-energy particles are not created on Earth. They can only be created in violent events, like exploding stars, collisions of celestial objects, or matter falling into a black hole. This means that if we observe these particles, they have travelled for ages before getting to us. They bring information with them from areas in the universe that otherwise we can’t see or know anything about. By observing cosmic showers, and piecing together their information, we can learn a lot about the influential events that help create and shape our universe. Our tiny, high-energy messengers will bring us closer to understanding the great unknown that is the cosmos.